Switching from liquefied petroleum gas to synthetic gas
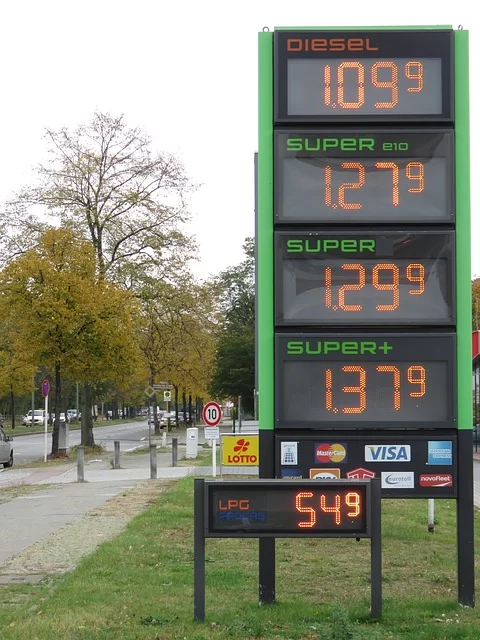
We used to like Liquefied Petroleum Gas
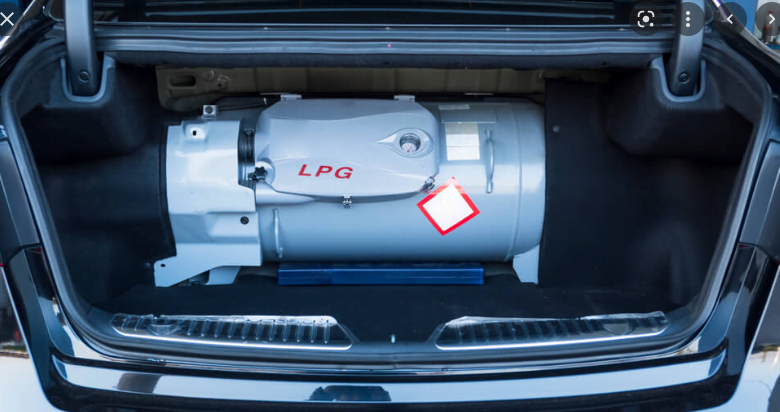
Liquefied Petroleum Gas is created when crude oil is refined or is extracted when natural gas is produced; either way, LPG is sourced from fossil fuels.
The components of fossil fuels are decaying plants and animals. These fuels may be burned to provide energy and can be found in the crust of the Earth. Fossil fuels include, for example, coal, oil, and natural gas.
LPG has a high caloric or heating value, meaning that it produces a lot of heat quickly when used as an energy source. Additionally, LPG virtually lacks sulphur, resulting in cleaner burning. Most Australian Taxis are fuelled by LPG, in fact the Australian Taxi Industry is one of the cleanest anywhere in the world.
So, LPG is excellent; it’s just that the manner we get it isn’t so great.
Is there an alternative to LPG that does not rely on fossil fuels?
If you read my page ‘Loads of Rubbish’ you’d know that I’m very excited about Sierra Energy’s FastOx Gasification Process as a way of stopping rubbish going to landfill as well as a way of making it the rubbish in the ground a very valuable resource, we can process it to make Syngas.
Syngas is a mixture of carbon monoxide and hydrogen, typically the Syngas is derived via gasification of solid feed-stocks such as coal or biomass or from natural gas.
Syngas can be utilised as a stand-alone fuel source for power generation or as an intermediary product in the chemical industry to produce synthetic natural gas, synthetic liquid petroleum, ammonia, and methanol.
Synthetic fuel, also known as Synfuel, is a liquid or gaseous fuel created from Syngas
Gas-to-liquids (GTL) is a technique that can convert Syngas into very clean-burning diesel fuel, liquid petroleum gas, base oil, and naphtha. The end products are colourless and odourless hydrocarbons with very low impurities
The most widely known GTL technology is Fischer-Tropsch process that converts Syngas into liquid fuels with the application of catalyst, heat and pressure.
The Internal Combustion Engine
One of the greatest inventions ever made by humans, the internal combustion engine is crucial to the generation of power, getting us to and from the grocery store and has quite a lot of uses.
Both stationary and moving machinery benefit greatly from its effectiveness. Compared to other combustion technologies, it offers a number of benefits, such as low capital costs, durability, strong part-load performance, high operational efficiency, adaptability, and generally safe operation.
Imagine if we switched from using gasoline to using Synfuel, which is produced from Syngas produced from your waste, to power these engines. What a fantastic scenario!
The internal combustion engine is very tolerant towards contaminants as compared to gas turbines.
We know that for sure because we know that the petrol we pump in from the Service Stations, isn’t always as clean a what we think it is.
For instance, my home country of Australia is one of just six OECD nations without any automobile emissions regulations. With a maximum of 150 parts per million (ppm) of sulphur for 91 octane normal unleaded and up to 50 ppm for our 95 and 98 premium unleaded, our fuel quality is amongst the filthiest in the world.
Typically, Petrol is made up from around 150 different hydrocarbons, including butane, isopentane, and pentane, benzene, toluene, ethylbenzene, and xylene. If you live in Australia, you can count on your petrol having around 15 times more sulphur in it than any other 1st world country.
What have we learnt so far?
What we do know is that the petrol driven internal combustion engines that we drive thousands of kilometres in each year can run rather well on pretty crappy fuel.
We know that they run very well and very clean on liquefied petroleum gas (LPG). In fact, LPG powered cars provide a number of environmental advantages, including cleaner breathing air for humans, lower CO2 emissions and lower particle emissions. Converting a vehicle to LPG contributes significantly to a greener earth.
There was a time in Australia where LPG dual fuel conversions were very popular and the government even subsidised the cost of getting your car changed over. The cost of LPG was about 25% of the cost of petrol. You don’t get the mileage you’re accustomed to with unleaded petrol but as long as the cost of LPG is half or less than unleaded, than it’s a very good idea.
Of course, we found lots of ways to ruin that and now LPG is nearly expensive as petrol.
But that’s ok, we know we have experience with converting our petrol driven vehicles to dual fuel LPG and we know that the Australian Govt did previously think it a good idea for people to drive around without polluting the planet.
So maybe all we need is another form of liquefied gas?
Maybe something that is incredibly clean?
What about a non fossil fuel source?
Heck, what if we could get that fuel source from the rubbish we’ve been burying in the ground for the last 100 years?
Imagine if, in addition to all of that, you lived in a country with some of the world’s smartest scientists.
I honestly don’t know why I’m not driving around in a vehicle powered by liquefied synthetic gas (LSG) today. What is going on Australia?
Folks, we either want to start caring for this world or we don’t; at some point, we must make a choice.
Create a plan and then stick to it; ideally, that plan will be aimed towards the welfare of this planet.
[…] https://www.healthisrock.com/liquefied-synthetic-gas-from-your-rubbish/ […]
[…] 8. Liquefied Synthetic Gas and petroleum gas […]
[…] 8. Liquefied Synthetic Gas and petroleum gas […]
[…] A car that runs on synthetic gas, that sounds crazy, doesn’t it? […]